UG103: How and why stars blow up
David Noel
<davidn@aoi.com.au>
Ben Franklin Centre for Theoretical Research
PO Box 27, Subiaco, WA 6008, Australia.
Stars have a definite lifetime
All the stars most familiar to us, those visible with the naked eye, lie in our home galaxy, the Milky Way. Around 1911, two astrophysicists, Ejnar Hertzsprung and Henry Norris Russell, created a very useful diagram of stars according to their colours and intensities.
This diagram is known as the Hertzsprung-Russell Diagram, or H-R Diagram.
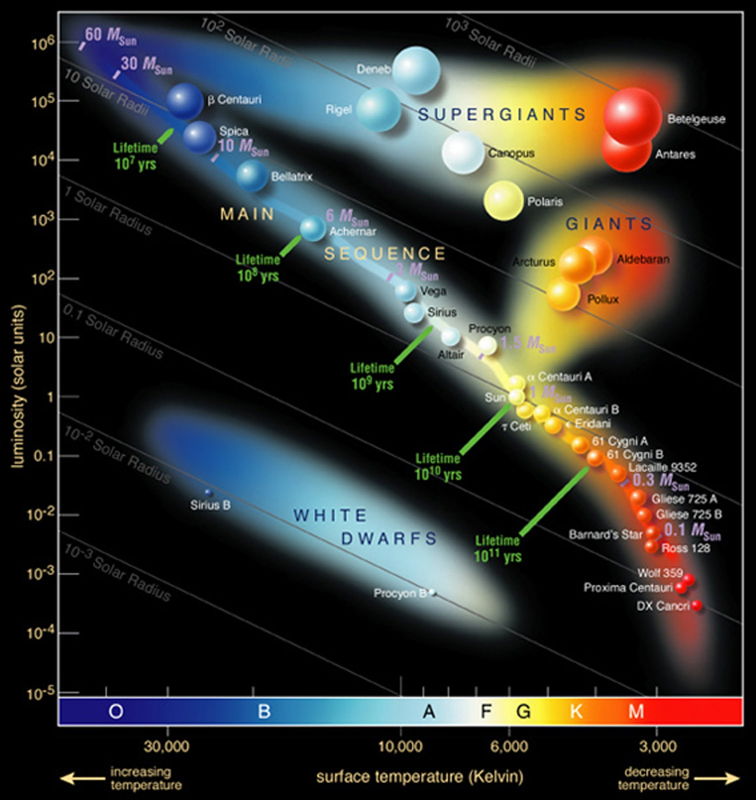
Fig. UG103-F1. The Hertzsprung-Russell Diagram. From [1].
Let's look at the stars on the Main Sequence of the Hertzsprung-Russell Diagram (Figure F1). It has been found that the colours, temperatures, masses, and lifetimes of these stars obey quite strict rules. A red star always has a low mass and intensity (compared to our Sun), a low temperature, and a long lifetime (again compared to our Sun). A blue star always has a large mass, a high temperature and intensity, and a short lifetime, again in comparison with the Sun.
These main-sequence stars can be called Fusion Stars. They produce light (and other electromagnetic radiation) as a consequence of nuclear reactions occurring within them, involving fusion of atomic nuclei. These reactions initially involve Hydrogen nuclei, and as the star ages, the nuclei of heavier atoms are involved -- first Helium, and then Carbon, Oxygen, Nitrogen, and so on up the atomic-weight scale.
We've noted that fusion stars have lifetimes in the inverse sense to their mass -- massive stars have short lifetimes, less-massive stars have longer ones. Bur what processes occur when a fusion star comes to the end of its life, and why?
The conventional idea is that fusion stars die when they run out of nuclear fuel. Here is one explanation, from [3].
"Stars die because they exhaust their nuclear fuel. The events at the end of a star's life depend on its mass. Really massive stars use up their hydrogen fuel quickly, but are hot enough to fuse heavier elements such as helium and carbon. Once there is no fuel left, the star collapses and the outer layers explode as a "supernova". What's left over after a supernova explosion is a "neutron star" -- the collapsed core of the star -- or, if there's sufficient mass, a black hole.
Average-sized stars (up to about 1.4 times the mass of the Sun) will die less dramatically. As their hydrogen is used up, they swell to become red giants, fusing helium in their cores, before shedding their outer layers, often forming a "planetary nebula". The star's core remains as a "white dwarf", which cools off over billions of years".
We will see that although the results of a star dying are well described in this passage, the reason for the events happening are quite wrong. Running out of hydrogen, or of any other element, is not the reason why stars blow up. Figure F2 is a graphic showing that the outcome when a star dies is dependent on its initial mass.
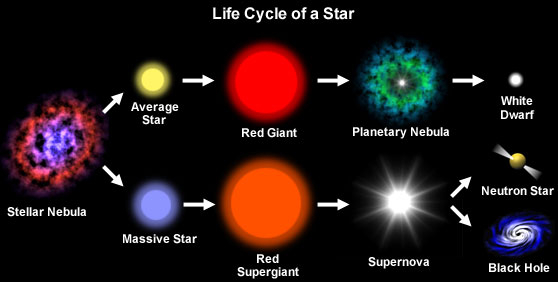
Fig. UG103-F2. Life Cycle of Stars. From [2].
This picture of the results when stars die is generally accepted and not in dispute here. But something not yet generally appreciated, is that the resulting objects are all objects in a range of "Vortex Stars", very similar rapidly rotating objects, differing only in their masses.
In fact, Vortex Stars are themselves subject to a strict set of rules concerning their masses, energy outputs, sizes, and rotation rates. With increasing mass, a vortex star rotates more quickly, and is of smaller radius (opposite to the familiar case). It also has a higher density.
White Dwarfs. Lower-mass Vortex Stars, falling in the "White Dwarf" category, rotate fast compared to fusion stars (the Sun takes about 27 days to rotate once). White Dwarfs have rotation rates of from several times per hour to as fast as once every 25 seconds..
Neutron Stars. Medium-mass Vortex Stars are called Neutron Stars, and are believed to consist almost entirely of compacted neutrons. They are denser than White Dwarfs and rotate more quickly -- from about 25 seconds down to a fraction of a second per rotation [5].
Black Holes and AGNs. Stellar Black Holes are left behind from the blowup of the highest-mass fusion stars. AGNs (or supermassive black holes) are formed by gravitational suction at the centres of galaxies, which are themselves a type of vortex, at a quite different scale. AGNs slowly accumulate both mass and rotational energy from the capture of both the vortex stars and the fusion stars around them. AGNs are known with rotation times approaching a thousandth of a second.
There is a more extensive writeup on Vortex Stars in
UG102: Understanding Vortex Stars -- White Dwarfs, Neutron Stars, Black Holes, and AGNs [4].
The importance of rotational energy in star blowup
Here the evidence is presented that star blowup is not due to running out of "nuclear fuel", but is a necessary consequence of changes in the bodies' rotation rates. If you look closely at the nuclear fuel idea, you will find that there is no evidence for it. Nor is there a plausible mechanism for hydrogen scarcity to cause a star to blow up.
In contrast, the rotational energy scenario gives a succinct and quantitative explanation for a star to split apart.
The standard Law of Conservation of Mass/Energy states that mass and energy are conserved, that is, they can be converted into other forms, but can be neither destroyed or created. The energy of rotation of a body, sometimes called angular momentum, is a form of energy, and is conserved.
This is nicely illustrated by looking at how an ice skater speeds up their rate of spin [6].
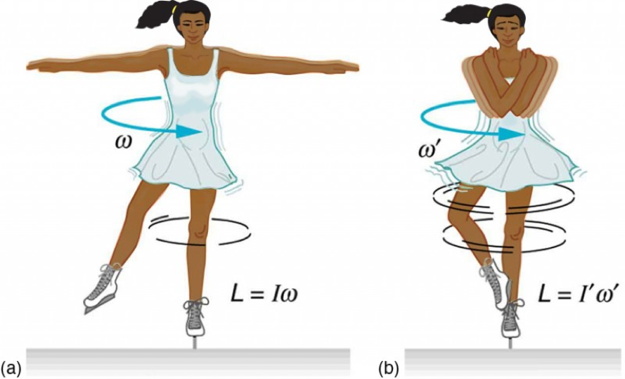
Fig. UG103-F3. How an ice skater speeds up spin rate. From [6].
"An ice skater is spinning on the tip of her skate with her arms extended. Her angular momentum is conserved because the net torque on her is negligibly small. Her rate of spin increases greatly when she pulls in her arms, decreasing her moment of inertia. The work she does to pull in her arms results in an increase in rotational kinetic energy".
When the skater has her arms extended, the rotational energy of her hands and outer arms is quite high, because these parts are most distant from her (vertical) axis of rotation. If she draws her hands in, their high rotational energy is transferred to the rest of her body, which in consequence speeds up.
The same principle operates with stars. If their radius is reduced, conserved rotational energy will cause the whole star to rotate more quickly.
What causes stars to reduce their radius?
In fusion stars, by definition they fuse lighter elements into heavier ones. Again, by definition heavier elements are more dense -- they contain the same mass in a smaller space. Fusion necessarily ends up with the original material more tightly packed.
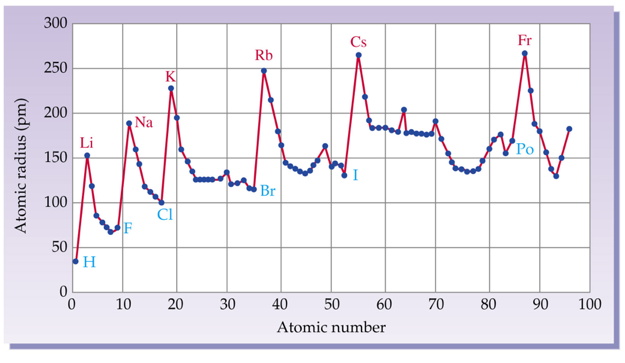
Fig. UG103-F4. Variation of atomic radius with atomic number. From [7].
Figure F4 shows a graph of how the sizes of atoms vary with their atomic number, that is, the number of protons their nucleus contains. It can be seen that Lithium (Li), which contains just three protons, has about the same radius as Uranium, which contains over 90. So the fusion process can shrink matter down to one-thirtieth of the size.
Under the immense pressures applying in the centres of stars, ordinary atoms can be compressed down into neutrons, which are essentially just atomic nuclei, and are immensely smaller -- smaller by a factor of trillions.
These huge compressions occur in the cores of stars, where the highest pressures are found. As the core gets smaller, conservation of rotational energy means that it must rotate more quickly. The more mass the star started off with, the more compressed its core becomes, and the faster it has to rotate.
And this is the clear reason why fusion stars blow up. Their Core takes on more and more rapid spin, until it can no longer maintain cohesion with the outer part of the star, which is flung off into space. This first looks a swelling transition star, such as a Red Giant, eventually its surface is stretched enough to break up into a planetary nebula.
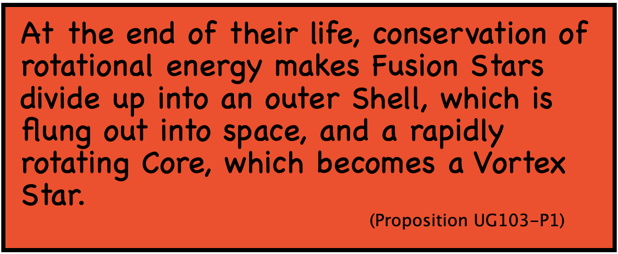
Proposition P1.
Once revealed, this explanation of fusion star blowup seems almost self-evident. And it does explain how Neutron Stars can come into existence -- they are the cores of medium-mass stars, left behind by blowup.
* * * * * * * * * * * * * * * * * * * *
References and Links
[1]. Hertzsprung-Russell diagram. https://en.wikipedia.org/wiki/Hertzsprung-Russell_diagram .
[2]. Astronomy/Stellar Evolution. https://scioly.org/wiki/index.php/Astronomy/Stellar_Evolution .
[3]. Alastair Gunn. How do stars die? http://lasp.colorado.edu/outerplanets/kbos_comets.php .
[4]. David Noel, UG102: Understanding Vortex Stars: White Dwarfs, Neutron Stars, Black Holes, and AGNs. http://www.aoi.com.au/UG/UG102/ .
[5]. Neutron star. https://en.wikipedia.org/wiki/Neutron_star .
[6]. Conservation of Angular Momentum. https://nigerianscholars.com/tutorials/rotational-motion-angular-momentum/conservation-of-angular-momentum/ .
[7]. Atomic Radius. https://sites.google.com/site/honorschemistryonline/the-periodic-table/2-main-trends.
Go to the UG Home Page
Compilation started 2022 Mar 15. First version on Web, 2022 Mar 22. V. 1.1, Adjusted 2025 May 29, Uploaded Ju 5..