XT806: What is Equatorial Forcing?
"XT806: What is Equatorial Forcing?" is an extract from an article about how solar systems are built from interstellar matter ("Oort Soup"). Once a central sun is built up, Equatorial Forcing is the mechanism by which planets and other bodies within the sun's gravitational reach are gradually forced into the sun's equatorial plane of rotation.
In this extract we look at the example of our own Solar System, with its planets and asteroids in the Inner System, and the bodies beyond the planets, such as dwarf planets in the Kuiper Belt, and beyond them, comets of the enclosing Oort Cloud. The Equatorial Forcing mechanism also acts on moons and rings around planets, as shown brilliantly in the case of Saturn's Rings, and even on whole galaxies, to shape them into galactic discs.
The story so far
Knowledge of our Solar System extends right back into remote human history. By the 1930s, the picture had been well established that the known planets orbited about the Sun in a disc-like plane, which roughly coincided with the plane of the Sun's equator.
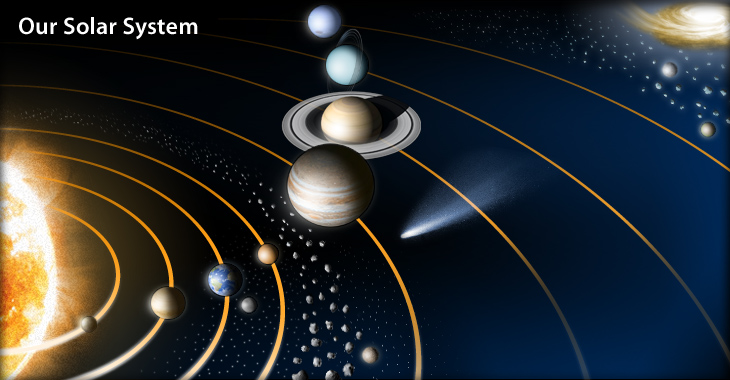
Figure CSM1. Our Solar System. From [3].
The plane in which the Sun's planets orbited was not a perfectly flat one, nor did it exactly match the equatorial plane of the Sun. The following table gives some relevant data.
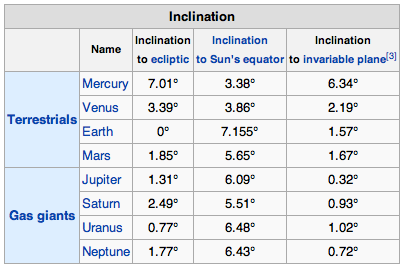
Figure CSM2. Inclinations of planetary orbits. From [6].
The table shows the names of the planets, followed by the angles between their planes of rotation and the ecliptic (the plane of rotation of Earth). Then there are the angles between the planetary planes and the the Sun's equatorial plane. The last column shows the angles between the planetary planes and the invariable plane (the weighted average of all planetary orbital and rotational planes).
It can be seen that the various planes differ by up to 7 degrees or so, not huge, but still significant.
Back in the 1930s, our knowledge of the Solar System was pretty well limited to the nine planets (then including Pluto), the asteroids (smaller bodies, mostly found in the Asteroid Belt between Mars and Jupiter), some comets, and a number of known moons of the planets. Mercury and Venus have no moons, the Earth has one, Mars two, and Jupiter, Saturn, Uranus, and Neptune were known to have a few each. That was the full extent of our knowledge.
Better telescopes, and rocket-powered space vehicles, have changed all that.
Our Solar System -- where we are today?
With increasing improvements in telescopes and in experimental techniques, we have come to know more about our own solar system, reaching out well beyond the orbits of Neptune and Pluto. Space probes sent to the Moon and out to the other planets yielded huge amounts of new, often surprising, information. More and more moons were found circling the outer planets, and more and more became known about the regions beyond Neptune.
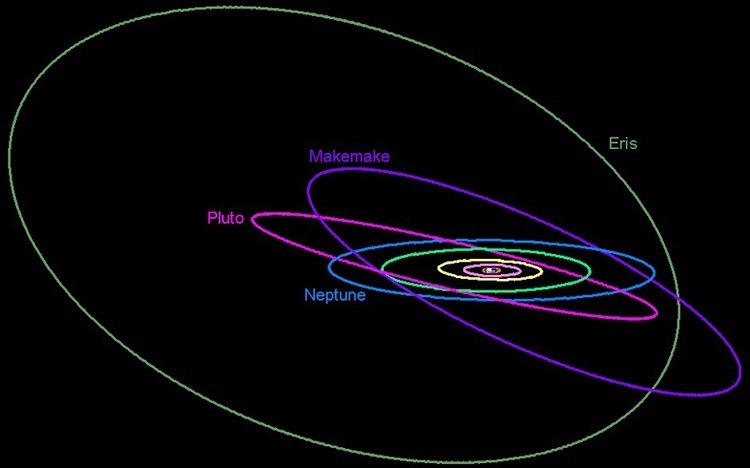
Figure CSM3. Tilting of planetary orbits. From [7].
The graphic shows the orbits of the planets, out to Neptune, lying in an approximate plane. Beyond Neptune, the orbits of dwarf planets such as Pluto and Eris are strongly tilted. The dwarf planets mostly lie in what's called the Kuiper Belt, a region beyond Neptune only investigated in recent decades.
The Kuiper Belt is usually represented as another disc containing relatively small planetary bodies, of the size of Pluto or less, orbiting the Sun beyond Neptune. Neptune orbits at 30 AU (Astronomical Units, the distance of Earth from the Sun), and the Kuiper Belt lies at 30-100 AU from the Sun [9, 11].
The Kuiper Belt [9] is known to contain over 1000 identified objects (KBOs), including at least three dwarf planets (Pluto, Haumea, and Makemake), More than 100,000 KBOs over 100 km in diameter are believed to exist. Originally considered a planet, Pluto's status as part of the Kuiper Belt caused it to be reclassified as a "dwarf planet" in 2006.
In more recent times, the outermost part of the Kuiper Belt, from 50 AU to 100 AU from the Sun, is often treated as a distinct entity, the Scattered Disc. Within the Scattered Disc lies the dwarf planet Eris, believed to be larger in diameter, and probably mass, than Pluto [10]. This makes it the ninth-largest Sun-orbiting body in the Solar System.
The trend in objects orbiting the Sun will be apparent. The orbits of the eight planets lie approximately on a plane (up to 7 degrees variation). In the inner Kuiper belt, deviations from this plane are much larger -- Pluto's orbit has an inclination of over 17 degrees to the ecliptic [13], and is also highly elliptical (non-circular). The dwarf planet Makemake has an orbit inclined at 29 degrees.
Fig. CSM4. Orbit of Pluto -- ecliptic view. This "side view" of Pluto's orbit (in red)
shows its large inclination to Earth's ecliptic orbital plane. From [11].
When it comes to Eris, in the outer Outer Kuiper Belt or Scattered Disc, its orbit's inclination is higher still, 44 degrees (see Fig. CSM3). So, the further out from the Sun, the more inclined the orbits.
According to [10], "Eris (minor-planet designation 136199 Eris) is the most massive known dwarf planet in the Solar System and the ninth-most-massive body known to directly orbit the Sun. It is estimated to be 2,326 km in diameter, and 27% more massive than Pluto, or about 0.27% of the Earth's mass.
Eris was discovered in January 2005 by a Palomar Observatory-based team led by Mike Brown, and its identity was verified later that year. It is a trans-Neptunian object (TNO) and a member of a high-eccentricity population known as the scattered disc. It has one known moon, Dysnomia. As of 2014, its distance from the Sun is 96.4 AU, roughly three times that of Pluto. With the exception of some comets, Eris and Dysnomia are currently the most distant known natural objects in the Solar System."
Beyond the Kuiper Belt
The region beyond the Kuiper Belt is called the Oort Cloud. In contrast to the Kuiper Belt, the Oort Cloud has no pretence of being based on a disc. Instead, it is spherical, like the very thick skin of a pomelo or grapefruit. It is also enormously more extensive than the regions looked at so far -- while the Kuiper Belt extends out to 100 AU, the Oort Cloud goes out to 100,000 AU [12].
This distance is almost 2 light-years, which is about half the distance to the nearest star. In a sense, the Oort Cloud is only a theoretical concept, a way to refer to distant bodies in space which are still within the Sun's gravitational influence. But the Sun's gravitational pull at these distances is weak indeed, and if this pull is the principal force acting on a body, its 'orbit' about the Sun may be highly elliptical and take thousands of years to complete one cycle.
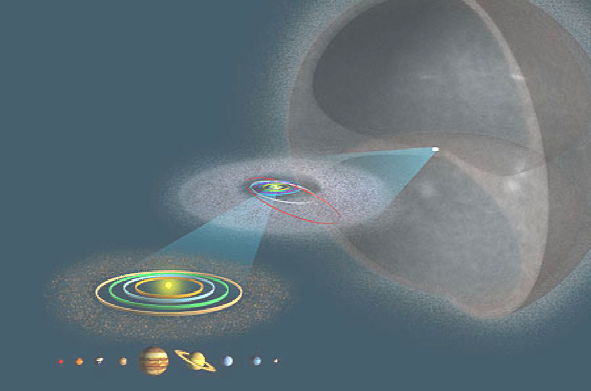
Figure CSM5. The Kuiper Belt and the Oort Cloud in the outer Solar System. From [8].
The Oort Cloud has most relevance to us as being the place where most comets appear to 'originate', that is, these 'long-period' comets may swoop in to the inner Solar System and be recorded there, before retreating back to where they came from. Because their times to complete one orbit are so long, maybe many thousands of years, we don't have any historical records of earlier appearances.
One reason why the Oort Cloud is treated as a sphere, rather than a disc, is because comets can appear from any direction, such as at 90 degrees to the plane of the ecliptic. Again, the further out from the Sun, the more inclined the orbits may be.
Here is how Nick Stroebel describes the situation [18].
"The Oort Cloud is a large spherical cloud with a radius from 50,000 to 100,000 AU surrounding the Sun, filled with billions to trillions of comets. It has not been directly observed. Its existence has been inferred from observations of long period comets. Long period comets have very elliptical orbits and come into the inner solar system from all different random angles (not just along ecliptic). Kepler's third law says that they have orbital periods of hundreds of thousands to millions of years.
However, their orbits are so elliptical that they spend only 2 to 4 years in the inner part of the solar system where the planets are, and most of their time at 50,000 to 100,000 AU. With such long orbital periods their presence in the inner solar system is, for all practical purposes, a one-time event. Yet we discover several long period comets every year. This implies the existence of a large reservoir of comets".
We do have historical records of some short-period comets, such as Halley's Comet. This is the best-known of the short-period comets, and is visible from Earth every 75 to 76 years. Halley is the only short-period comet that is clearly visible to the naked eye from Earth. Halley, like many comets, follows a retrograde orbit, that is, it orbits the Sun in the opposite direction to the planets.
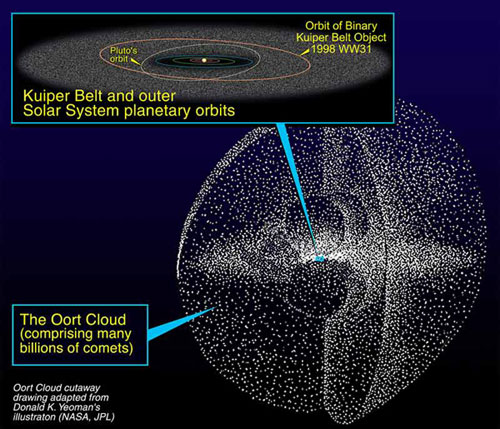
Figure CSM6. The Oort Cloud, warehouse for billions of long-period comets. From [16].
We can record the orbit of a long-period comet quite accurately, and so could calculate when it should next appear. But even then, because comets are typically of low mass, they are easily affected by the gravity of other Solar-System bodies, which could well throw out their orbits.
Bigger bodies within the Oort Cloud
Existing telescopes and techniques can only detect Oort-Cloud objects having orbits or paths which bring them fairly close to the inner solar system.
There was much excitement in 2003 when Sedna, a dwarf planet with an unusual orbit, was detected at 90 AU, three times as far from the Sun as Neptune. Sedna never comes much closer to us -- it has a highly elliptical orbit, taking about 11,400 years per cycle, from a maximum of 937 AU to a minimum of 76 AU [21].
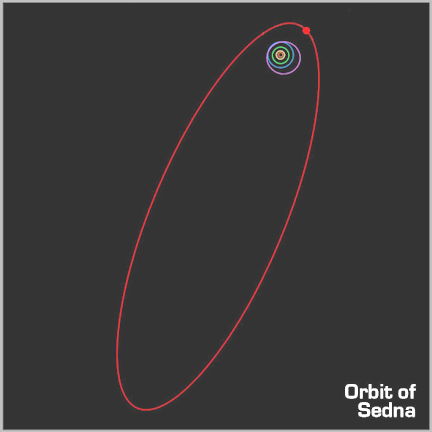
Figure CSM7. The orbit of Sedna, an Oort Cloud object. From [20].
Spectroscopy has revealed that Sedna's surface composition is similar to that of some other trans-Neptunian objects, being largely a mixture of water, methane and nitrogen ices with tholins. Its surface is one of the reddest in the Solar System. Sedna's exceptionally long and elongated orbit, taking approximately 11,400 years to complete, and distant point of closest approach to the Sun, at 76 AU, have led to much speculation about its origin.
Mike Browne, one of the discoverers of Sedna, realized that Sedna was something new for astronomical theory. He wrote "Seven years ago, I knew with certainty that the discovery of Sedna in a strange orbit that never brought it close to any planet was telling us something profound about our solar system. I also knew that Sedna would never divulge her secrets alone. To learn more, we'd have to find more things like Sedna".
Sedna was the first dwarf planet detected which can be classed as a member of the Oort cloud. It has been estimated that another 40 to 120 Sedna-sized objects exist within this region [21]. As of end 2013, four currently known objects (90377 Sedna, 2000 CR105, 2006 SQ372, and 2008 KV42) are considered members of the inner Oort cloud [6].
The most-recently discovered of these, 2008 KV42, nicknamed Drac (short for Dracula), is a trans-Neptunian object orbiting the Sun in retrograde motion (backwards) and almost perpendicular to the ecliptic: it has a 104-degree inclination [15].
In [15] it also says "The orbits of trans-Neptunian objects provide important clues as to how the outer Solar System took form and evolved. Discoveries of new classes of objects have led to fresh insights into the early history of the Solar System, challenging accepted theories. The discovery of 2008 KV42, the first-ever object in this region to be detected with a retrograde orbit, promises to do just that."
Dr JJ Kavelaars of the National Research Council of Canada has noted [15] that "Although we've been specifically looking for highly-tilted trans-Neptunians for some time now, we didn't expect to find a retrograde one. A number of theories on the formation of the outer Solar System have suggested that such things might be out there, but observational searches for them are very difficult."
What's the makeup of the Oort Cloud?
There is an important thought to be drawn from the orbit of Sedna (Fig. CSM7). Firstly, this dwarf planet never comes very close to the Inner Solar System (where the planets dwell). Secondly, its orbit is highly elliptical.
The thing is, Sedna would never have been detected with current techniques, unless it was on an elliptical orbit which happens to have brought it comparatively close to us in recent years. So, only Oort-Cloud objects with very elliptical orbits are detectable for us.
What about other Oort-Cloud objects with more circular orbits? They are likely to be undetectable at present. So there could well be a huge reservoir of such objects which we do not know about.
Moreover, further out in the hypothetical Oort Cloud, near the gravity watershed between our Sun's gravitational influence and that of the next star, the forces of gravity acting there are very weak. Objects sitting there are probably not in anything we would describe as an orbit, but are just free-floating.
Let's try for another conclusion, from a plot of comet orbit inclinations.
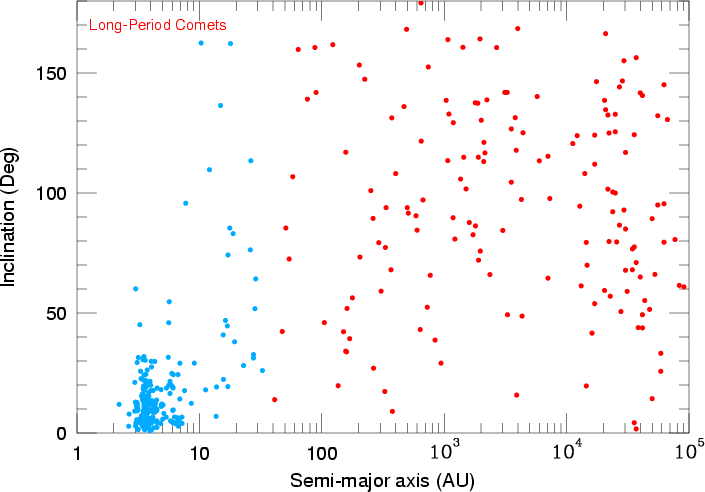
Figure CSM8. Inclinations of long-period comets. From [17].
The plot shows Inclination (tilt of the comet's orbit compared to the plane on which the planets lie) plotted against Semi-major axis (distance from the Sun to the furthest point on the comet's orbit).
From the plot it is obvious that comets which orbit closer to the Sun (up to about 10 AU) have low inclinations, mostly under 30 degrees. Beyond about 100 AU (the end of the Kuiper Belt and the start of the Oort Cloud), inclinations are essentially random -- comets come from any given direction.
On this plot, an inclination over 90 degrees means one tilted beyond the vertical. This is another way of saying that the comet is retrograde, orbiting in the opposite direction to the planets. So an orbit inclination of, say, 105 degrees is the same as an inclination of 75 degrees, except that the comet runs along its orbit in the reverse direction (called retrograde). The conclusion is that Oort Cloud has no particular structure, but is approximately the same everywhere.
Another important event in the early history of the Solar System was the Late Heavy Bombardment. Here is how [37] characterizes it.
"The Late Heavy Bombardment is a hypothetical event thought to have occurred approximately 4.1 to 3.8 billion years ago. During this interval, a disproportionately large number of asteroids apparently collided with the early terrestrial planets in the inner solar system, including Mercury, Venus, Earth and Mars. The LHB happened "late" in the Solar System's accretion period when the Earth and other rocky planets formed and accreted most of their mass; it is a period still early in the history of the solar system as a whole."
Why do the Solar-System planets lie in a plane?
We've already seen that in the early days of the Solar System, the mass of planets and other planetesimals within the gravitational influence of the Sun may have followed every sort of orbit or movement possible, and may have undergone collisions or aggregations, or added their mass to that of the Sun.
To achieve the current disc-like distribution of the planets, there must have been forces which altered or amalgamated all these random orbits into the nice flat disc we see today. It appears that this was due to gravitational forces from the Sun, very slowly altering these orbits to conform with the equatorial plane of rotation of the Sun.
Proposition CSMP9
This idea ties in well with the Late Heavy Bombardment event of 3.8-4.1 billion years ago. If true, it means that the "straightening-out" or regularization of the orbits of the planets mostly happened around 500 million years after the Solar System first formed.
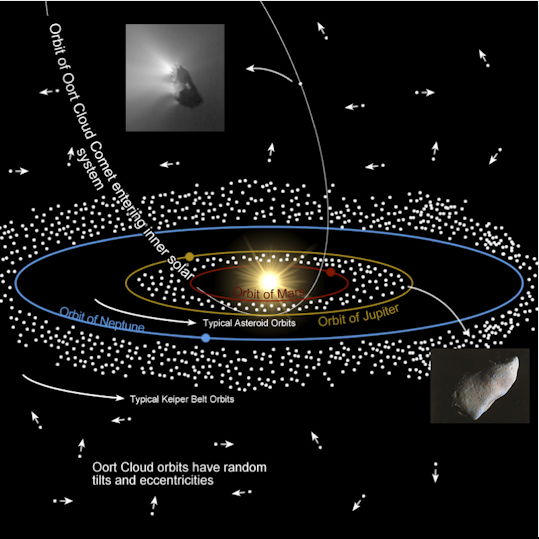
Figure CSM13. Flattening of orbits into a plane. From [38].
The concept also ties in well with the increasing inclinations of orbits of comets and dwarf planets -- the further they are from the Sun, the weaker its gravitational influence.
One of the modern requirements for a Sun-orbiting body to be classed as a planet is that it has cleared the area around its orbit of other significant bodies. In the same way, it might be said that for a part of space to be classed as a solar system, the central sun or suns must have cleared their section of the local Oort Cloud.
It will be remembered that our solar-system planets do not move exactly in a plane, but vary in their inclination to the Sun's equator by up to 7 degrees or so. This deviation can be ascribed to the slow pace of orbit flattening, taking up to billions of years, and its weakening with distance from the Sun. Also it is likely that Jupiter, easily the most massive of the planets, might be the most resistant. All 5 planets from Mars outward have inclinations only half a degree or so different to Jupiter's.
This concept of flattening of planetary orbits is not the current conventional view, and so few mathematical analyses of the gravitational interactions involved have been done. Let us move on now to an analogous situation which is much better researched.
The Rings of Saturn
The Rings surrounding the planet Saturn are one of the most beautiful and interesting sights in the Solar System. They are also one of the earliest discoveries, first noted by Galileo in 1610.
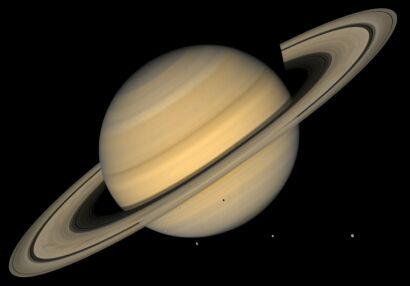
Figure CSM14. Saturn. From [39].
Here is a summary from Wikipedia [41].
"The rings of Saturn are the most extensive planetary ring system of any planet in the Solar System. They consist of countless small particles, ranging in size from micrometres to metres, that orbit about Saturn. The ring particles are made almost entirely of water ice, with a trace component of rocky material. There is still no consensus as to their mechanism of formation".
The dense main rings extend from 7,000 km to 80,000 km above Saturn's equator (Saturn's equatorial radius is 60,300 km). With an estimated local thickness of as little as 10 metres they are composed of 99.9 percent pure water ice with a smattering of impurities. The main rings are primarily composed of particles ranging in size from 1 centimetre to 10 metres."
The rings are not continuous, instead they have gaps and thin sections, which create divisions. The rings were named in order of discovery, using capital letters of the alphabet, starting with 'A'.
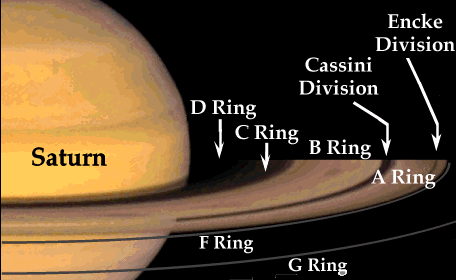
Figure CSM15. Divisions in the rings of Saturn. From [40].
The rings are the flattest known objects in the Solar System. With a width up to 80,000 km and a thickness of 10 metres, they are 8 million times wider than thick. Saturn's Rings orbit exactly above Saturn's equator [42], the inclination of the ring particles' orbits to the equator is zero degrees.
The other three outer planets, Jupiter, Uranus, and Neptune, also have small ring systems, and these rotate above their planet's equators also.
A lot of mathematical analysis has gone into working out how the rings operate. They are actually quite complex, some rings having 'shepherd moons' moving within them, other rings affected by a phenomenon called 'resonance' with Saturn's major moons. But clearly the forces involved create a very stable overall situation.
Whatever the underlying mechanism, these examples prove without any doubt that orbit-flattening forces have been active to produce and maintain these amazing structures. The exceptional flatness of the ring structures, taking orbit-flattening to the max, may be due to the small masses of the component particles compared to the masses of their planets.
* * * * * * * * * * * * * * * * * * * * * * * *
Extract from P1: The Cosmic Smog model for solar system formation, and the nature of 'Dark Matter'.
References and Links
3. Solar System Exploration. http://solarsystem.nasa.gov/planets/ .
6. Orbital Inclination. http://en.wikipedia.org/wiki/Orbital_inclination .
7. Cosmology. http://journalofcosmology.com/Cosmology8.html .
8. Outer Solar System. http://deepspaceexplorers.org/ .
9. Kuiper belt. http://en.wikipedia.org/wiki/Kuiper_belt .
10. Eris (dwarf planet). http://en.wikipedia.org/wiki/Eris_(dwarf_planet) .
11. Oort cloud. http://en.wikipedia.org/wiki/Oort_cloud .
12. Comets -- Target Earth. Video. http://www.youtube.com/watch?v=4i4pYPI8OQs .
13. Pluto. http://en.wikipedia.org/wiki/Pluto .
15. 2008 KV42. http://en.wikipedia.org/wiki/2008_KV42 .
16. The Oort Cloud. http://upload.wikimedia.org/wikipedia/commons/0/03/Kuiper_oort.jpg .
17. Orbit of Sedna. http://upload.wikimedia.org/wikipedia/commons/d/d9/Sedna-PIA05569-crop.jpg .
18. Nick Stroebel. Comet Orbits---Oort Cloud and Kuiper Belt. http://www.astronomynotes.com/solfluf/s8.htm .
20. Long-period comets. http://www.boulder.swri.edu/~hal/talks/oort/CIW/oort020.html
21. 90377 Sedna. http://en.wikipedia.org/wiki/90377_Sedna .
37. Late Heavy Bombardment. http://en.wikipedia.org/wiki/Late_Heavy_Bombardment .
38. A Background in Asteroids, Comets and NEOs. https://lcogt.net/education/article/background-asteroids-comets-and-neos .
39. Saturn. http://www.solstation.com/stars/saturn0.jpg
40. Astro 1: `Slides' for Class 39 - The Jovian Planets and Pluto. Astro 1: `Slides' for Class 39 - The Jovian Planets and Pluto.
41. Rings of Saturn. http://en.wikipedia.org/wiki/Rings_of_Saturn .
42. What is the angle between the plane of Saturn's rings and its equatorial plane? http://zombal.com/zomb/scientific-question/what-is-the-angle-between-the-plane-of-saturns-rings-and-its-equatorial-plane .
Go to the AOI Home Page
Latest version on Web, 2017 Jan 12