UG102: Understanding Vortex Stars --
White Dwarfs, Neutron Stars, Black Holes, and AGNs
David Noel
<davidn@aoi.com.au>
Ben Franklin Centre for Theoretical Research
PO Box 27, Subiaco, WA 6008, Australia.
The two main star classes -- Fusion and Vortex stars
Observations over past centuries, using instruments such as telescopes and spectrographs. has give us a fairly good knowledge of the different types of stars and their properties.
One of the best guides to understanding these properties can be had using a chart developed in the early 1900s, called the Hertzsprung-Russell Diagram (Figure F1).
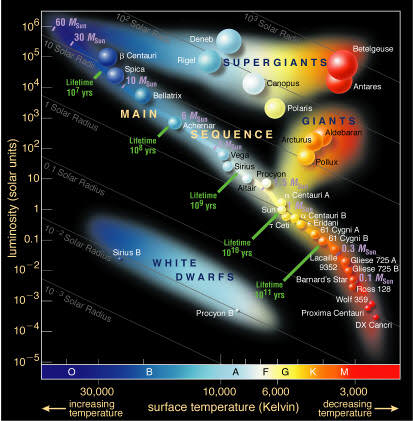
Figure UG102-F1. The Hertzsprung-Russell Diagram. From [5].
In this diagram, the vertical scale gives the luminosity (intrinsic brightness) of a star, and the horizontal scale shows its temperature, which for various reasons is also its colour -- hotter stars are bluer, cooler ones are redder.
This diagram is based on stars in our own galaxy, the Milky Way. The long band from top left to bottom right (labelled "Main Sequence") includes all the "normal" stars. These range from the most massive violet ones, with 60 times the mass of our Sun, down to the tiny dull red ones, with about 7.5 % of the solar mass.
All these stars produce their energy from nuclear fusion -- fusing hydrogen into helium, then into carbon, nitrogen, oxygen, and heavier atoms still. Here these stars are called "Fusion Stars". There is a lot more information in the Hertzsprung-Russell diagram, such as the stars' lifetimes --more massive stars burn out quickly, tiny ones last much longer. There is also a good Youtube video, giving more detail, at [4].
In the lower left part of the H-R diagram is a band labelled "White Dwarfs". These are the least massive of a quite different sort of star, the "Vortex Stars". We'll go on to explain this other class of star.
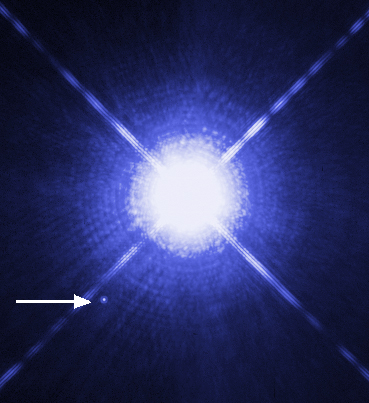
Figure UG102-F2. Sirius A and B. From [6].
Binary stars, where two stars rotate around a common centre of gravity, are quite common in the Universe. In some cases, one star of the pair is a fusion star, the other a vortex star, as in Figure F2. This is an image of the stars Sirius A and Sirius B taken by the Hubble Space Telescope. Sirius B, which is a white dwarf, can be seen as a faint point of light to the lower left of the much brighter Sirius A.
In the top right corner of the H-R diagram are bands labelled "Supergiants" and "Giants". Below we'll look at the evolution of fusion stars through their lifetimes, and see how all go through an expansion or explosion phase as they cease fusion processes, and become vortex stars. The bands labelled Giants include "Transition Stars" -- stars which are in the middle of this change process.
The evolution of Fusion Stars
Fusion stars, like most space objects, start off from the aggregation of interstellar gases and dust under gravitational forces. As evolution of a star proceeds, how it appears depends on the mass of the particular aggregation.
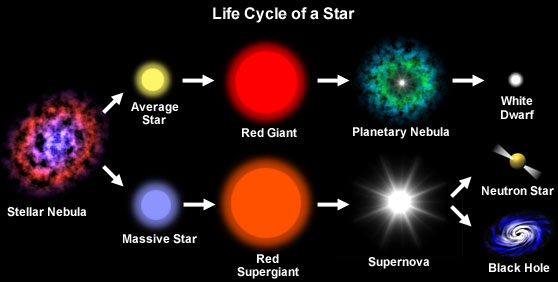
Figure UG102-F3. Life cycles of stars. From [6].
As mentioned above, the lifetime of a star in its fusion phase depends on its mass. For an average star such as our Sun, the lifetime is around 10 billion years -- we are currently close to halfway in the process. Very massive fusion stars may last only 10 million years or less, while tiny red dwarfs can live in their fusion phase for hundreds of billions of years.
Eventually, for all fusion stars, fusion ceases (the star "runs out of fuel"), and the star undergoes an expansion or explosion process which splits the outer shell of the star from its core. The outer, rapidly-expanding part is a sphere of material, which is not in itself generating energy (although it may be passing on energy received from the core), and may amount to half, or more, of the original fusion star's material.
We recognise the expanding shell as a red giant or supergiant, and eventually, as its material becomes more widely stretched, as a planetary or other nebula. Because the object is a transition star, undergoing fairly rapid change, it may show measurable changes in size, brightness, and temperature. The bigger the mass of the original fusion star, the more rapid the transition process -- for what are called supernovas, the expansion is rapid enough to be called an explosion, and may last only a matter of weeks.
The core becomes a Vortex Star
The core or inner part left after expansion or explosion of a fusion star is here called a Vortex Star. Names applied depend on the mass of the star -- the lowest grade usually recognized is a White Dwarf, from a fusion star a few times the mass of the Sun or less.
If the original star had a mass between about 10 and 25 times the Sun's mass, the core remnant is called a neutron star. For original masses higher than that, the resulting object is called a stellar-mass black hole.
All these objects can be classed as Vortex Stars. All consist of very rapidly rotating, highly massive objects which have the properties of vortexes, including the ability to draw matter in.
In addition, all vortex stars emit beams of energy, and in some cases beams of matter, along their spin axes. They therefore exist in a state of dynamic equilibrium, absorbing matter and energy from their surroundings, and emitting it along their axial beams.
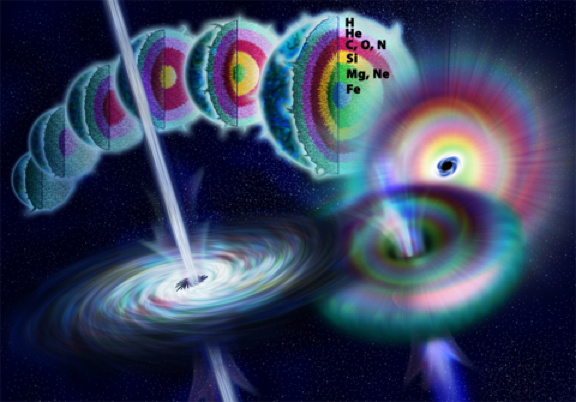
Figure UG102-F4. Star evolution concept. From [1].
Figure F4 is an artist's conception of how a fusion star evolves into a vortex star. It's shown as a rapidly-rotating disc. There is no direct evidence that such a disc exists, but it gives a good visual image to grasp.
As well as emerging from a dying fusion star, a vortex star may be formed through merger of many stars, vortex and fusion alike. These mergers can form an AGN (Active Galactic Nucleus) or supermassive black hole, such as exists at the heart of every galaxy.
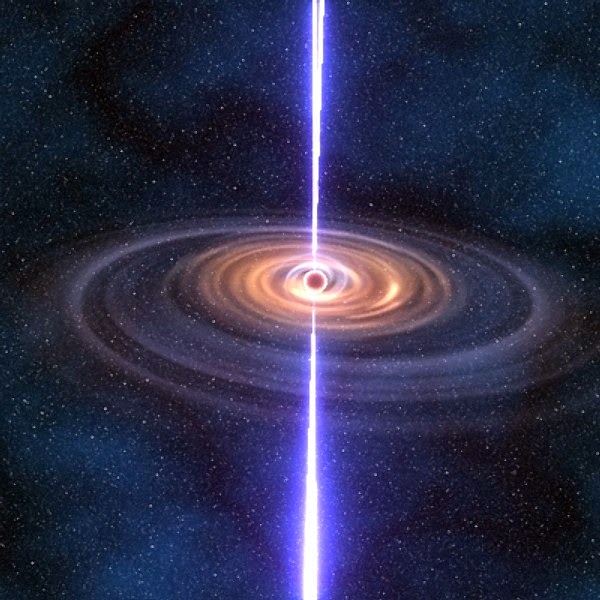
Figure UG102-F5. Artist's conception of a pulsar vortex star. From [7].
These AGNs are identified by a whole range of names, according to their properties, such as pulsars, quasars, Seyfert galaxies, blazars, radio galaxies, and magnetars. All emit tight beams of radiation along their axes. Sometimes the name used reflects their orientation with the observer.
According to [8], the difference between Quasar, radio galaxy and a Blazar is the angle of the stream. If the stream is straight up, it is a radio galaxy and we are not in the firing line. If the stream is angled slightly towards us, then it's a Quasar, and if the stream is angled directly towards us, it's a Blazar.
The importance of orientation when viewing a Vortex Star
Because Vortex Stars concentrate their radiation output into two tight beams, it's vital to distinguish between Fusion and Vortex stars when considering the amount of energy they put out.
Normal Fusion stars radiate fairly uniformly in all directions, so their apparent brightness falls off with with the square of their distance. This factor is taken into account when estimating how far away stars lie, from their brightness (with two stars of similar intrinsic brightness, the one twice as far away will have one-quarter of the apparent brightness).
With a Vortex star, this relationship no longer holds. Since the star's outputs are confined to narrow beams, its radiation only reduces slightly with distance, as the beam spreads a little. This applies when the beam is viewed head-on. When viewed at an angle, the vortex star is only visible at all when its beam hits dust or gas in its path.
This has led to serious misunderstandings of energy outputs when a Vortex star is assumed to be a Fusion star. For example, White Dwarfs, such as the star Sirius B shown in Figure F2, are often assumed to have low radiation outputs, whereas in most cases it is just that their axial beams are not pointed directly at the observer.
In another example, stars producing what are called Fast Radio Bursts are all actually the AGNs of very distant galaxies which happen to be radiating directly toward the observer. Assumptions that they are Fusion stars leads to hugely exaggerated ideas of their outputs.
Vortex Stars may be very dense
According to [2], a White Dwarf may have a density of 1 x 109 kg/m3, a billion times denser than water. A neutron star may come in at from 8.4 x 1016 to 1 x 1018 times that of water, trillions of trillions times denser. Small black holes come in denser still, at around 2 x 1030 kg/m3.
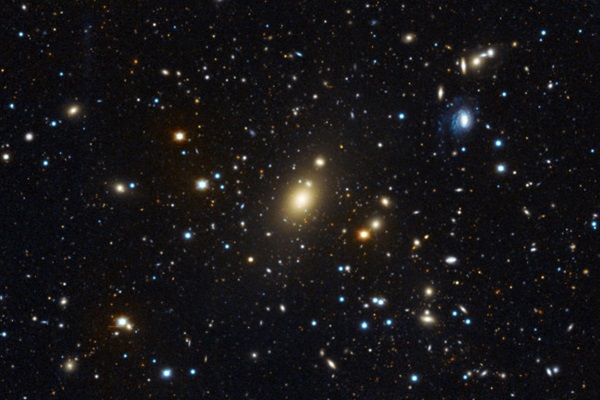
Figure UG102-F6. The Abell 85 galaxy cluster. From [3].
According to [3], the Abell 85 galaxy cluster is home to the largest black hole known in the universe. This monster black hole packs the mass of 40 billion suns.
These figures are hard to visualize. Some idea of the enormous densities involved can be had by considering the size of our Earth if it was made of neutron-star material, or of black-hole material, as in Figure F7.
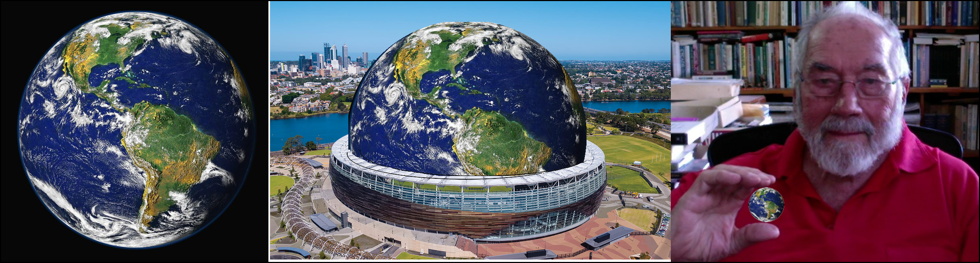
Figure UG102-F7. The Earth built from different materials.
Our Earth has a diameter of about 12,740 kilometres. If made from neutron-star material, it would be about 330 metres across, and would fit in Perth's Optus Stadium. If made from black-hole material, it would have a diameter of about 3 centimetres.
Properties of Vortexes
Vortex Stars, as with all vortexes, have an important property -- they try to suck in material from outside.
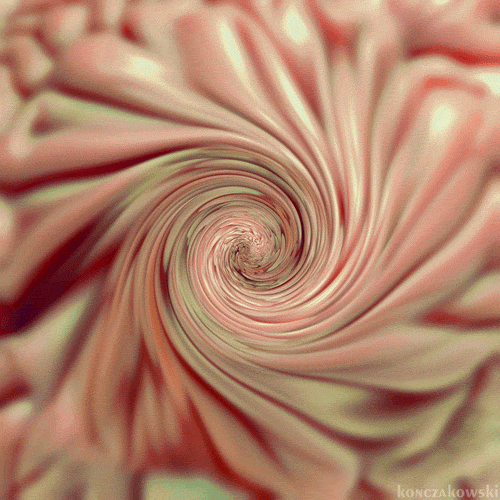
Figure UG102-F8. A vortex sucking in material. From [9].
As well as the normal vortex properties, Vortex Stars have the additional property of emitting radiation, and sometimes matter, along their axes. They therefore exist in a state of dynamic equilibrium.
Sitting at the centres of galaxies, AGNs continually exert a force called Spin Gravity, which tries to draw in material from intergalactic space as long as it can. In this way, each AGN gains matter, and some energy, from its surroundings.
At the same time, the AGN is losing energy, and sometimes material, through its axial beams. The more massive the AGN gets, the more energy it loses. The lifetime of the AGN depends on how the dynamic balance shifts.
There is more on Spin Gravity at Mass Gravity and Spin Gravity [10].
There is a detailed treatment of vortexes at The Spindle Vortex Model for Gravity, Energy, Matter, Magnetism, Antimatter [9].
* * * * * * * * * * * * * * * * * *
References and Links
[1]. Gamma ray burst. https://commons.wikimedia.org/wiki/File:Gamma_ray_burst.jpg .
[2]. White dwarf. https://en.wikipedia.org/wiki/White_dwarf .
[3]. Erika K. Carlson. This huge galaxy has the biggest black hole ever measured. https://astronomy.com/news/2019/12/this-huge-galaxy-has-the-biggest-black-hole-ever-measured .
[4]. Stars and Galaxies: The Hertzsprung-Russell Diagram
https://www.youtube.com/watch?v=_EtlJCfaxdc .
[5]. Fraser Cain. Hertzsprung-Russell diagram. https://www.universetoday.com/39974/hertzsprung-russell-diagram/ .
[6]. Astronomy/Stellar Evolution. https://scioly.org/wiki/index.php/Astronomy/Stellar_Evolution .
[7]. Tibi Puiu. Peculiar pulsar slows down before "glitching". https://www.zmescience.com/science/peculiar-pulsar-slows-down-before-glitching/ .
[8]. What are Quasars and Blazars and whats the difference?. https://www.universeguide.com/fact/quasar .
[9]. David Noel. BS802: GEMMA -- The Spindle Vortex Model for Gravity, Energy, Matter, Magnetism, Antimatter. http://aoi.com.au/BaseScience/BS802-Vortex/ .
[10]. David Noel. BS806: Mass Gravity and Spin Gravity -- Adjusting the Universe. http://www.aoi.com.au/BaseScience/BS806/index.htm .
Go to the Cosmology Plus Home Page
Compilation started 2021 Apr 6. First version 1.0 on Web 2021 Apr 9.